Combining various observational data of neutron-star collisions with nuclear physics calculations, an international team of scientists, including University of Minnesota Twin Cities researchers, has made a breakthrough in discovering the mysteries of what is inside neutron stars-formed when certain types of stars die in supernova explosions. Studying this dense matter gives new insight into measuring the expansion rate of our Universe.
Their results are published in the journal Science, a premier peer-reviewed academic journal of the American Association for the Advancement of Science.
In modern astrophysics, scientists combine different types of signals from space, in particular light, cosmic particles, and gravitational waves, to answer fundamental questions of cosmic history. This method of multi-messenger astronomy is a rapidly growing field. The "cosmic messengers" in the electromagnetic spectrum of light include gamma rays, ultraviolet, visible, infrared, and radio. Cosmic particles can be electrons, protons, neutrinos, complex atomic nuclei, and more. Finally, gravitational waves are tiny ripples in the fabric of space-time itself generated by accelerated masses such as neutron stars or black holes, which emerge from dying stars after the end of their lives.
Colliding neutron stars produce most of the heavy elements in the periodic table, and they allow for measuring the expansion rate of our Universe. By studying colliding neutron stars, astronomers can depict the properties of matter at very high densities, exceeding the density inside atomic nuclei-matter for which a single teaspoon of material would weigh an inconceivable several million tons. Black holes are the only thing denser, but they have so fully escaped the bounds of normal physics that they are not matter anymore.
To unravel the mysteries of the underlying physical processes during neutron star collisions, researchers from the United States, Germany, the Netherlands, Sweden, and France incorporated observations of neutron-star collisions with these signals both across the full electromagnetic spectrum and in gravitational waves.
"This work constitutes the most extensive analysis to date of the famous gravitational wave named GW170817 discovered in 2017. With contributions from nuclear physics, gravitational-wave astrophysics, optical and gamma-ray astronomy, we address two fundamental problems in physics today-the expansion rate of the Universe and equation of state of neutron stars," said Michael Coughlin, a University of Minnesota Twin Cities physics and astronomy assistant professor and co-author of the study. Coughlin led the optical data analysis and generation of the surrogate kilonova models.
The research team developed an interdisciplinary framework combining the observations with theoretical nuclear physics calculations to extract astrophysical information on these systems and matter under extreme conditions.
"With our method, we were able to constrain the size of a typical neutron star to be about 12 kilometers (a neutron star is an object with the size of a single city), but with a mass several hundred thousand times the mass of the Earth," said Tim Dietrich, a professor of? theoretical astrophysics at the University of Potsdam in Germany.
Further, the team used the extracted astrophysical information to determine the "Hubble constant," a fundamental constant that describes the expansion of the Universe.
"In the last few years, the scientific community tried to resolve tension among different determinations of the expansion rate of our Universe," said Ingo Tews, staff scientist at Los Alamos National Laboratory and co-author of the study. "Our new framework allowed us to re-measure the Hubble constant, and the final results show a mild preference for the previous Cosmic Microwave Background measurement."
Starting from theoretical input that describes the nuclear matter in the core of neutron stars, the researchers analyzed astronomical data in a multi-step procedure.
"We included neutron-star mass measurements through radio observations, the observation of a rapidly spinning neutron star, and observations of neutron star mergers via gravitational waves and electromagnetic signatures," Dietrich explains. "For the latter, we scanned the entire frequency spectrum ranging from the radio band to gamma-rays."
The developed framework is general and can easily be extended to detect more multi-messenger signals in the coming years, he said.
To read the research paper entitled "Multi-messenger constraints on the neutron-star equation of state and the Hubble constant," visit the Science website.
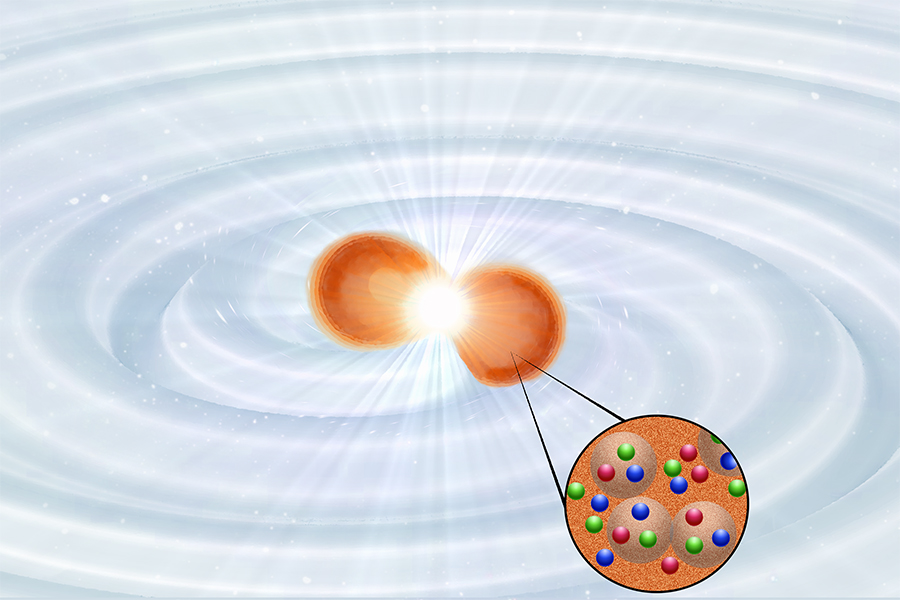