When you put your hand out the window of a moving car, you feel a force pushing against you called drag . This force opposes a moving vehicle, and it's part of the reason why your car naturally slows to a stop if you take your foot off the gas pedal. But drag doesn't just slow down cars.
Author
- Piyush Mehta
Associate Professor of Space Systems, West Virginia University
Aerospace engineers are working on using the drag force in space to develop more fuel-efficient spacecraft and missions, deorbit spacecraft without creating as much space junk , and even place probes in orbit around other planets .
Space is not a complete vacuum − at least not all of it. Earth's atmosphere gets thinner with altitude , but it has enough air to impart a force of drag on orbiting spacecraft, even up to about 620 miles (1,000 kilometers).
As an aerospace engineering professor , I study how drag affects the movement of spacecraft in orbit. Aerobraking , as the name suggests, is a type of maneuver that uses the thin air in space to apply a drag force in the direction opposite to a spacecraft's motion, much like braking in a car.
Changing an orbit
In space, aerobraking can change the orbit of a spacecraft while minimizing the use of its propulsion system and fuel.
Spacecraft that orbit around Earth do so in two types of orbits : circular and elliptical. In a circular orbit , the spacecraft is always at the same distance from the center of the Earth. As a result, it's always moving at the same speed. An elliptical orbit is stretched, so the distance from Earth − and the speed the craft moves at − changes as the spacecraft travels along the orbit.
The closest point in an elliptical orbit around Earth, where the satellite or spacecraft is moving fastest, is called the perigee . The farthest point, where it's moving slowest, is called the apogee .
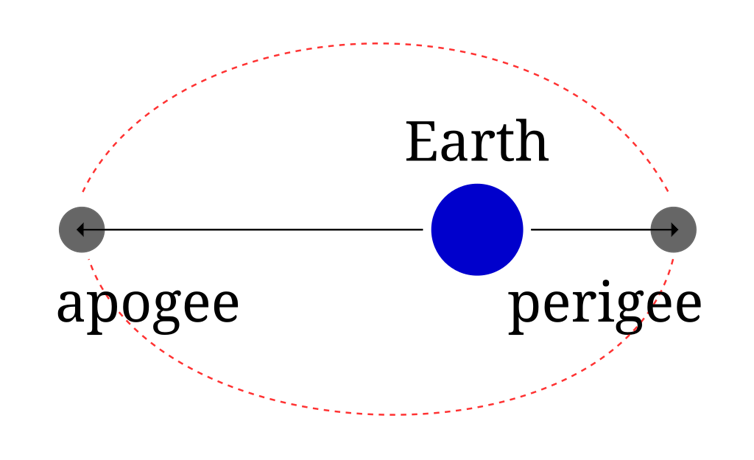
The general idea behind aerobraking is to start in a large circular orbit and maneuver the spacecraft into a highly elliptical orbit, so that the lowest point in the orbit − the perigree − lies in the denser part of the upper atmosphere. For Earth, that's between about 62 and 310 miles (100 and 500 kilometers), with the choice depending on time required to complete the orbit change.
As the spacecraft passes through this lowest point, the air exerts a drag force on it, which reduces the stretch of the orbit over time. This force pulls the craft toward a circular orbit smaller than the original orbit.
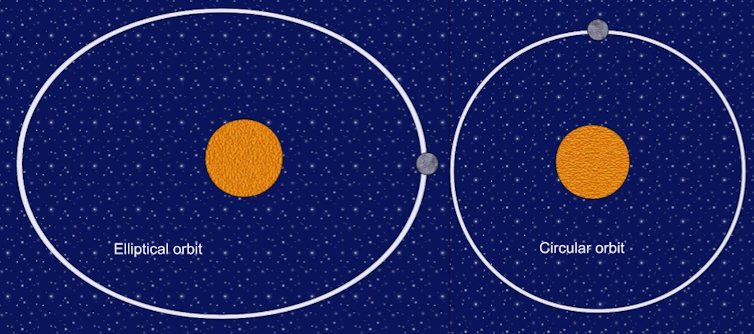
The first maneuver to put the spacecraft in an elliptical orbit so that drag can take effect does require using a propulsion system and some fuel. But once it's in the elliptical orbit, drag from the atmosphere slows the craft, and it doesn't need to use much, if any, fuel.
Aerobraking brings a craft from a large orbit to a small orbit and is not reversible − it can't increase the size of an orbit. Increasing the size of an orbit or raising the spacecraft to a higher orbit requires propulsion and fuel.
Aerobraking uses
A common case where spacecraft controllers use aerobraking is when changing the craft's orbit from a geostationary orbit − GEO − to a low Earth orbit , LEO. A GEO orbit is a circular orbit with an altitude of roughly 22,236 miles (35,786 km). In GEO, the spacecraft makes one orbit around Earth in 24 hours, so the spacecraft always stays above the same point on Earth's surface.
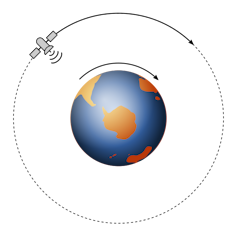
Before aerobraking, the spacecraft's onboard propulsion system thrusts in the opposite direction of the GEO orbit's motion. This thrust puts it into an elliptical orbit. The craft passes through the atmosphere multiple times, which eventually circularizes the orbit.
Once it makes it to LEO, the spacecraft may need to use a little bit of fuel to propel itself up into its target orbit. Usually, the lowest point of the original elliptical orbit is lower than the final target circular orbit.
This process is conceptually similar to how the U.S. Space Force's X-37B used aerobraking in early 2025 .
The U.S. Space Force reported that its unmanned spaceplane, X-37B , used aerobraking. This test demonstrated the craft's agility and maneuverability.
Another application for aerobraking is to make a spacecraft deorbit − or reenter the atmosphere − after it has stopped working. This way, the company or agency can dispose of the spacecraft and avoid creating space junk , since it will burn up in the lower atmosphere.
Aerobraking for interplanetary missions
A few Mars missions, including the Mars reconnaissance orbiter and the Mars Odyssey orbiter , have used aerobraking to reach their target orbits around the red planet.
For interplanetary missions like these, scientists use aerobraking in conjunction with the craft's onboard propulsion system. When a spacecraft arrives at Mars, it does so in a hyperbolic orbit .
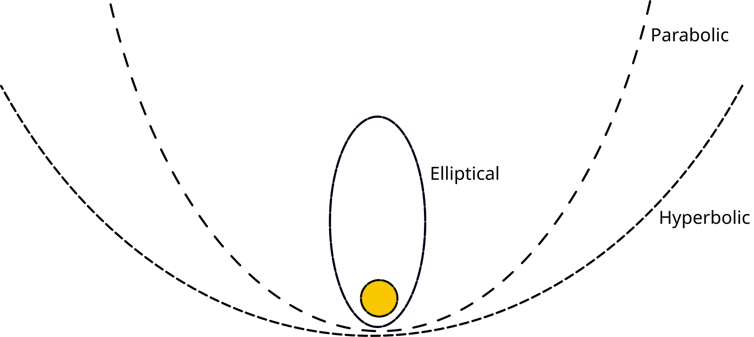
Unlike a circular or an elliptical orbit, the spacecraft's path in hyperbolic orbit won't keep it orbiting around Mars. Instead, it would fly through and depart Mars − unless it uses thrust from its propulsion system to get "captured" into a closed elliptical orbit.
As the spacecraft arrives at Mars, the onboard propulsion system fires to provide the force necessary to capture the spacecraft into a highly elliptical orbit around Mars. Once captured, scientists use aerobraking over several orbital passes through the atmosphere to achieve the final orbit, generally a circular one.
Aerobraking maneuvers can result in significant fuel savings. As humans get closer to landing on the surface of the red planet , the fuel savings enabled by aerobraking could save mass and allow each spacecraft headed to Mars to take more supplies.
In the grand arc of space exploration, aerobraking is not just a maneuver. It has a crucial role to play in the future of space operations and planetary missions and colonization.
Piyush Mehta receives funding from multiple federal agencies - NASA, NSF, NOAA, IARPA, and DoD.