Princeton's vital research across the spectrum of environmental issues is today and will continue to be pivotal to solving some of humanity's toughest problems. Our impact is built on a long, deep, broad legacy of personal commitment, intellectual leadership, perseverance and innovation. This article is part of a series to present the sweep of Princeton's environmental excellence over the past half-century.
Enter the front doors of Guyot Hall, the 111-year-old building that houses the Department of Geosciences at Princeton. Pass the glass specimen cases and the lobby's iconic model of planet Earth and head to room M56. There, beyond the rows of heavy-duty snow boots and bulky parkas, stands a walk-in freezer storing some of the rarest artifacts of modern climate science: ancient ice cores harvested from Antarctica. At more than 2 million years old, these are the oldest ice cores ever collected.
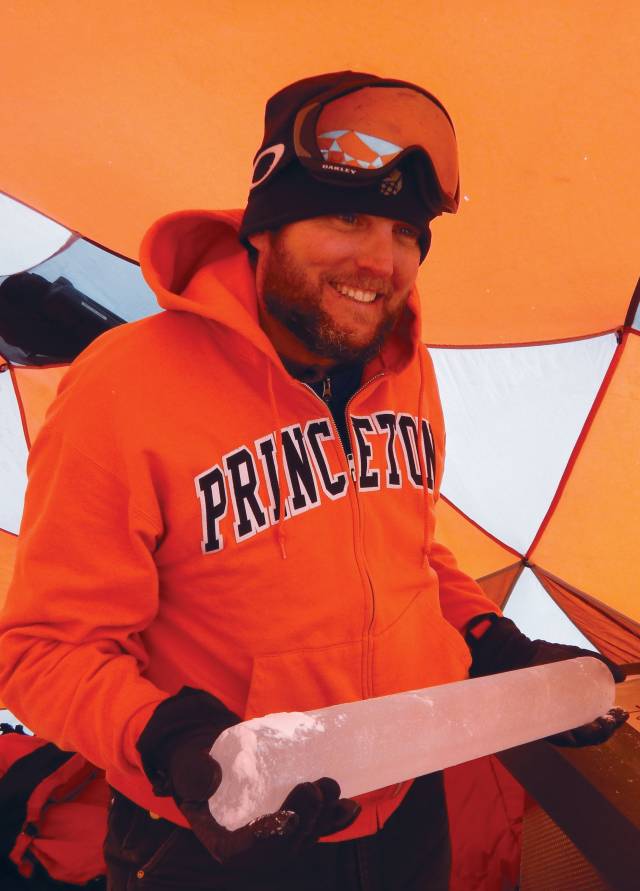
John Higgins
"Over the last 60-plus years, ice cores have produced the best evidence we have that carbon dioxide is linked to the Earth's climate" said John Higgins, project leader for the group that recovered the ice in 2019 and an associate professor of geosciences.
"When we had an ice age, atmospheric carbon dioxide was significantly lower than it is today, and every time we didn't have an ice age, atmospheric carbon dioxide was high - all that is known from ice cores," he said. "My team is contributing to that puzzle by extending that record further back in time."
The ice core research is emblematic of Princeton's approach to climate science across the board over the last half-century, said Bess Ward, the William J. Sinclair Professor of Geosciences and the Princeton Environmental Institute (PEI) and chair of the geosciences department. "There's a saying that geoscientists believe firmly, which is, 'The key to the present is the past, and the key to the future is the present,'" she said. "If we can understand the past, we can understand the future."
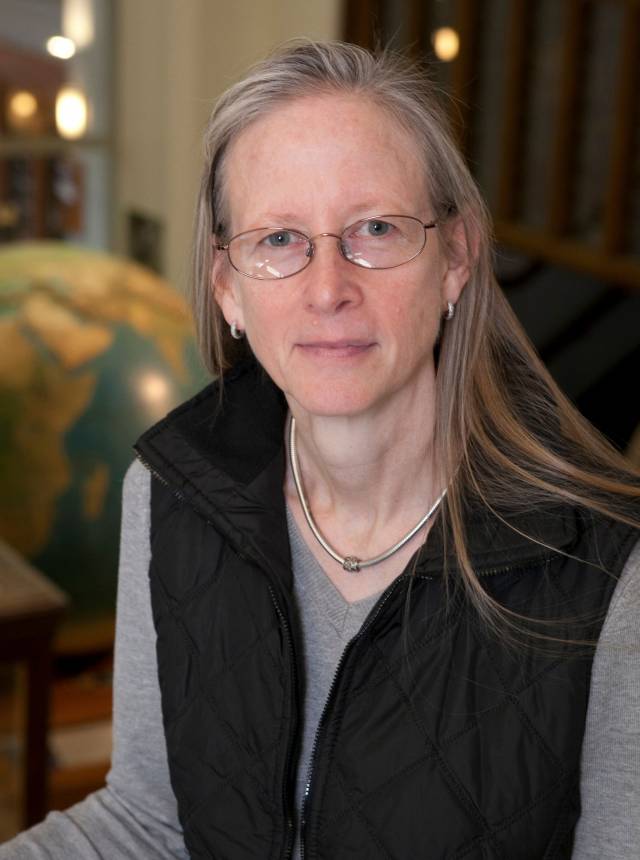
Bess Ward
And for more than 50 years, Princeton researchers have been doing just that. They've pushed back the boundaries of climate knowledge across a wide range of lynchpin issues. Princeton climate modelers, for example, developed the world's first coupled ocean-atmosphere model, using physical laws and present Earth conditions to develop mathematical algorithms that can predict how Earth's climate will respond to different conditions in the future - and to understand what drove climate changes in the past. Funneling data into the models are Princeton oceanographers and field geologists who have fanned out across the globe to understand what oceans and ecosystems are doing today. And paleoclimatologists have been using fossils, pollen records, ice cores and other tools to study how the global climate has already changed in the planet's long history.
Ground-truthing the theory
The combination of theory and observation has been critical. The importance of "boots on the ground, boats in the water" can't be overstated, said Gabriel Vecchi, a leading climate modeler as well as a professor of geosciences and PEI. Modern climate modelers such as himself benefit from the direct observations gathered by the geologists and oceanographers working "just down the hall," he said.
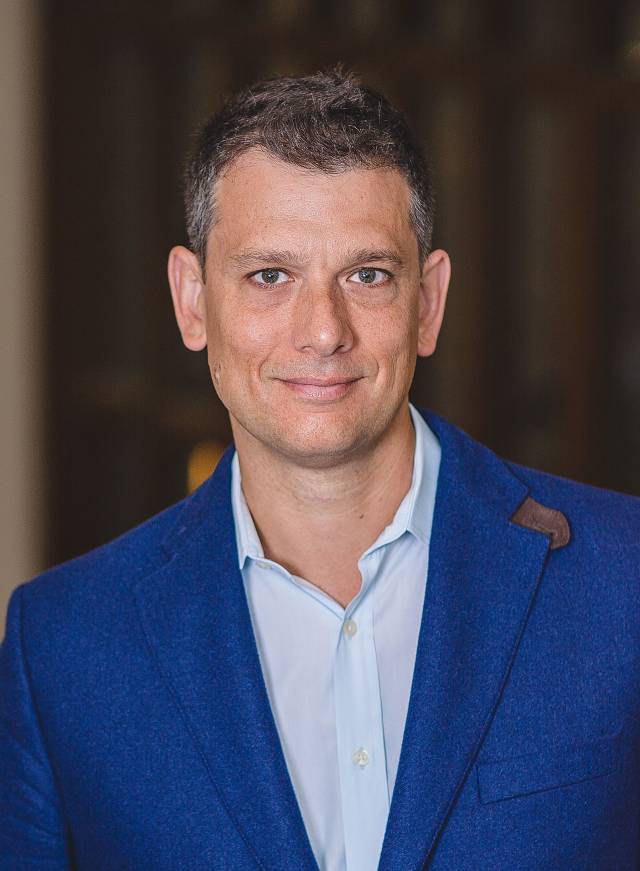
Gabriel Vecchi
"We can have great theories that make very nice predictions, but we need to test those predictions, and you only test those predictions with observations," Vecchi said. "At the same time, the fundamental processes that we put into our models have to be developed from some sort of empirical base. So, both on the front end and the back end of building models, you need to have very strong involvement of observations."
One Princeton scholar deeply involved in both theory and observation is Samuel G.H. "George" Philander, best known for his work on tropical oceans. His discovery of the recurring La Niña weather pattern and his seminal work on the related El Niño phenomenon dramatically improved scientists' understanding of those enormous climate fluctuations. That knowledge, in turn, helps governmental and economic planners prepare for their effects.
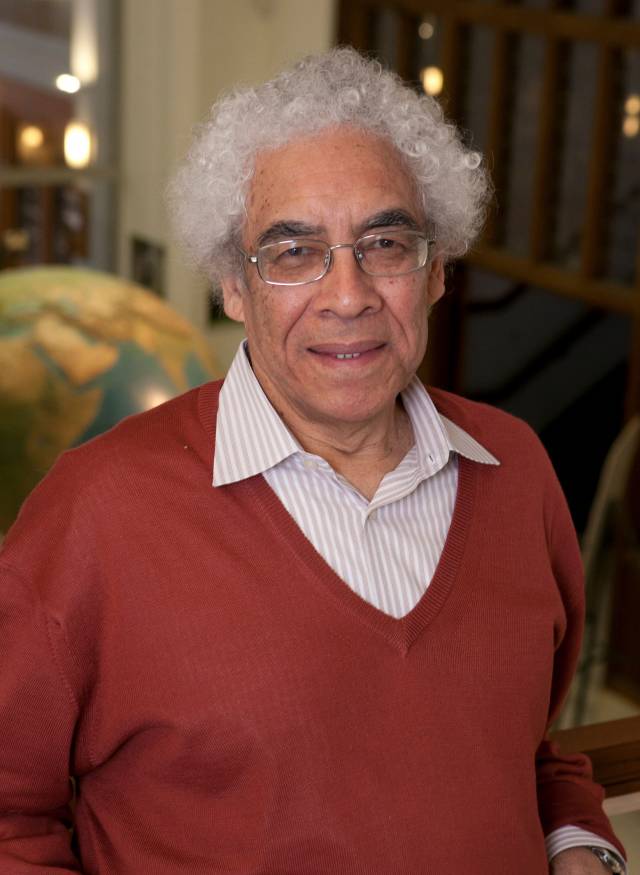
Samuel G.H. "George" Philander
Philander, now the Knox Taylor Professor of Geosciences, Emeritus, also helped organize a decade-long (1985-1994) international observational program known as TOGA, or Tropical Ocean Global Atmosphere. TOGA was designed to test the emerging theory of what is called "the coupled ocean-atmosphere system" in the tropics and paved the way for future ocean observation systems. It also led to improved simulations in global climate models.
"George was fundamental in developing our basic understanding of the El Niño phenomenon, which is the largest year-to-year fluctuation in the climate system," said Vecchi. For hundreds of years, El Niño had been known as an ocean phenomenon that warmed the seas off the coast of Peru and shifted rainfall and other climate patterns. Philander helped shift scientists' understanding of it from a purely oceanic event to one that was dependent on the coupled ocean-atmosphere system - the planet's interconnected and interdependent water and air.
"That was a fundamental shift in the way that we looked at these fluids," said Vecchi. "We realized that the ocean and atmosphere had to be understood together."
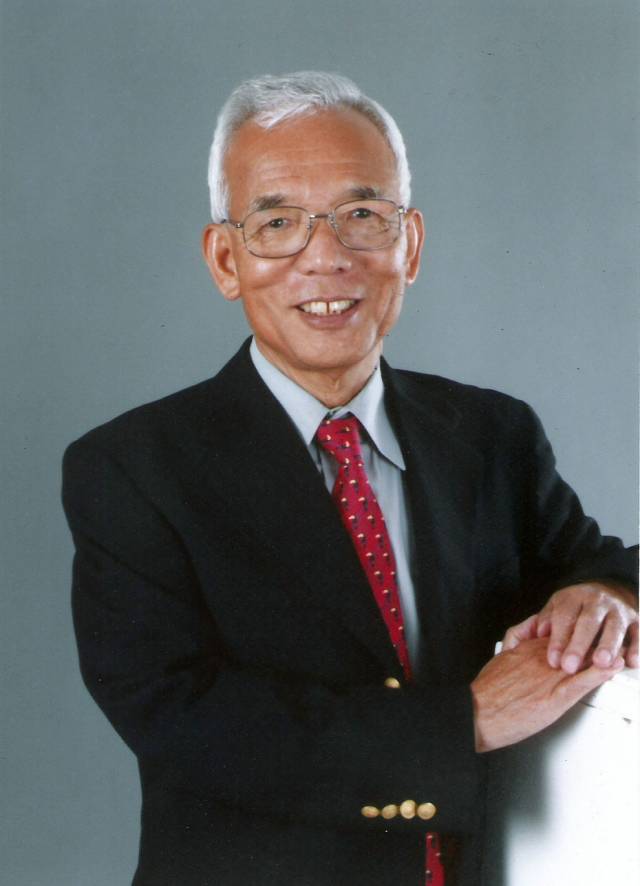
Syukuro Manabe
Also critical to that understanding was Syukuro "Suki" Manabe, one of the founders of modern climate modeling, who created the first coupled ocean-atmosphere computer model in 1969 with his colleague Kirk Bryan, an oceanographer. Both Manabe and Bryan were lecturers with the rank of professor at Princeton while also holding positions at the Geophysical and Fluid Dynamics Laboratory (GFDL) on Princeton's Forrestal Campus. "An improved version of that coupled model has become indispensable not only for predicting the climate change of the industrial present but also for exploring the climate of the geological past," Manabe said.
By changing the conditions (i.e., distribution of continent, concentration of carbon dioxide) modelers can recreate past eras as they predict the future, Manabe explained. Testing climate models against paleoclimates - ancient climates - is one of the key ways that climate modelers test their algorithms.
Frozen in time
The ice cores in Guyot Hall include many samples in addition to the multi-million-year-old record setter. The long, narrow cylinders of glacial ice, painstakingly gathered over the past half-century, are speckled with tiny bubbles of air that are trapped in the ice like dragonflies in amber. How do these trapped air bubbles form? As snow falls, it creates air pockets, and as that snow compacts into ice, those pockets become time capsules holding ancient air, whatever gases were present in the atmosphere, and even microscopic particles of pollen or volcanic ash - all clues to the past climate.
"For somebody who's trying to reconstruct what the environment was like in the past, you can't ask for anything better than a sample of ancient air, or a sample of ancient water," said Higgins, who keeps breaking