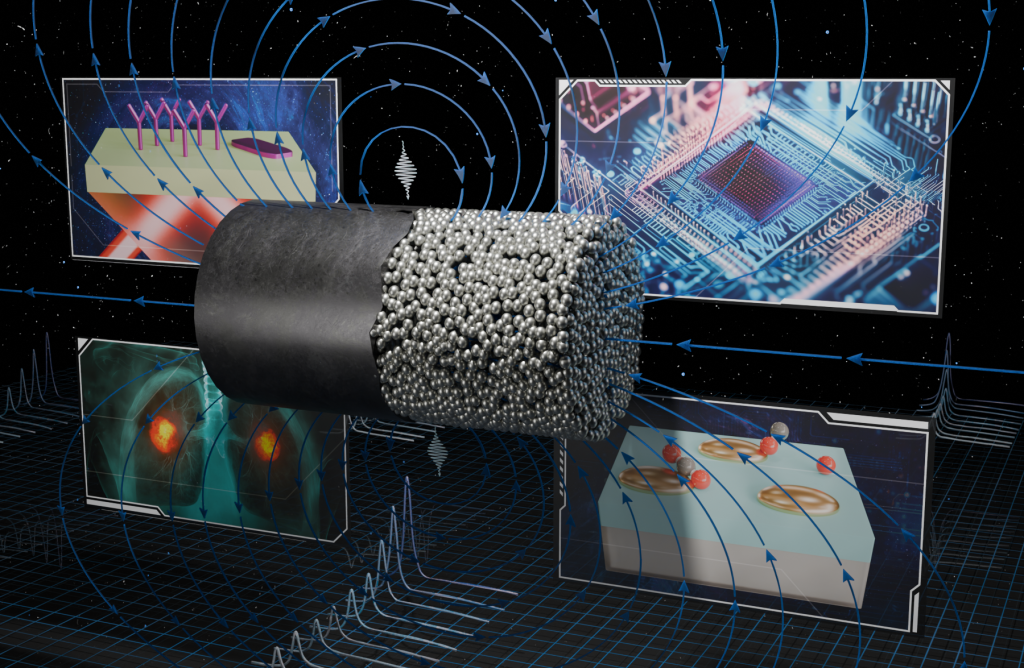
A research team from the Department of Energy's Oak Ridge National Laboratory, in collaboration with North Carolina State University, has developed a simulation capable of predicting how tens of thousands of electrons move in materials in real time, or natural time rather than compute time.
The project reflects a longstanding partnership between ORNL and NCSU, combining ORNL's expertise in time-dependent quantum methods with NCSU's advanced quantum simulation platform developed under the leadership of Professor Jerry Bernholc.
Using the Oak Ridge Leadership Computing Facility's Frontier supercomputer, the world's first to break the exascale barrier, the research team developed a real-time, time-dependent density functional theory, or RT-TDDFT, capability within the open-source Real-space Multigrid, or RMG, code to model systems of up to 24,000 electrons. Real-time, time-dependent describes the real-time evolution of the wavefunction or quantum-mechanical property. 24,000 electrons is about the same size as treating 4,000 carbon atoms or 2,400 water molecules treating the time evolution of all their electrons.
This research, led by ORNL's Jacek Jakowski and Panchapakesan Ganesh, is vital in designing new technologies such as advanced photovoltaic cells and emerging information systems.
These findings were published in the Journal of Chemical Theory and Computation .
"By directly observing thousands of electrons in real-time, we gain powerful insights into how materials respond at the quantum level," Jakowski said.
Metallic nanoparticles, or metals with dimensions within 1-100 nanometers, have unique optical properties caused by the way thousands of electrons within these metals interact with incoming light. It's critical for researchers to understand the ways these electrons move under a range of conditions to advance these new technologies.
The challenge in moving these technologies forward has been capturing these ultrafast electron dynamics in realistic nanoscale materials, or materials where at least one dimension is on the scale of nanometers.
This achievement enables the design of novel materials with tunable optical, electronic and magnetic properties and opens the door to new innovations in optical and quantum information devices.
RT-TDDFT is a quantum mechanical method that allows researchers to simulate how electrons move and interact in materials over time, once they are excited by an external stimulus. It works by calculating how the electron density in materials changes in response to the application of electric and electromagnetic fields (i.e. light), for instance.
"Think of it like watching a slow-motion replay of all the electrons in a tiny piece of metal responding to a flash of light, but at an incredibly detailed, quantum level," said Jakowski. "Our calculations are so large that they require one of the world's fastest supercomputers to run them in 'real time'. By capturing these electron movements at scale, we can predict how new materials will behave, potentially leading to more efficient photovoltaic cells, faster computers and better quantum technologies."
The RMG code developed by NCSU is a computational tool specifically designed to handle these simulations efficiently on DOE's exascale machines such as Frontier. It uses a flexible grid structure to represent electron behavior in real space, or 3D Euclidean space, allowing it to scale across such powerful supercomputers. Because RMG is open-source, other researchers can access, modify and apply the code to study a wide range of materials and physical phenomena.
Working in collaboration with NCSU and its platform for quantum-mechanical simulations, Jakowski, Ganesh and their team partnered through DOE's Innovative and Novel Computational Impact on Theory and Experiment, or INCITE, awards. Jakowski stressed that this achievement allows researchers to simulate and understand electron behavior in materials at realistic scales, enabling rapid tracking of electronic phenomena like plasmonic resonances and photoabsorption spectra.
Next steps for the project include simulating even more complex scenarios to discover new physics in quantum systems and enhancing efficiency and accuracy to handle larger, more intricate simulations.
"These developments hold great promise for creating novel devices with tailored electronic, optical and magnetic properties," said Professor Bernholc. "Ultimately, we hope our real-time approach will guide experimental efforts and accelerate breakthroughs in areas ranging from spintronics to quantum information science."
Primary funding for this effort was provided by ORNL's Center for Nanophase Materials Sciences. It was also supported by an INCITE allocation from the Department of Energy, which provided access to the Frontier supercomputer.
UT-Battelle manages ORNL for the Department of Energy's Office of Science, the single largest supporter of basic research in the physical sciences in the United States. The Office of Science is working to address some of the most pressing challenges of our time. For more information, please visit energy.gov/science . - Mark Alewine