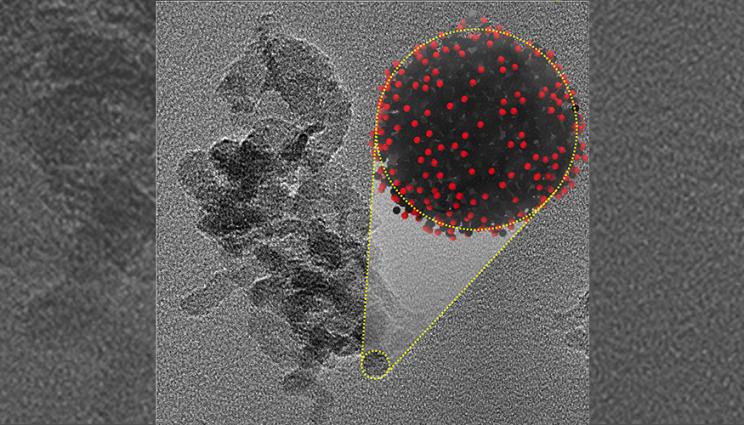
Nanocarbon condensate produced by ultrafast shock compression of liquid carbon monoxide and predicted by MD simulations. Lab research could open new avenues for nanocarbon synthesis.
Nanocarbons have emerged as major nanomaterials due to their many practical applications, including their potential use in energy conversion/storage, bioimaging, drug delivery, sensors, diagnostics and composites.
However, their synthesis remains poorly understood. A common high-pressure synthesis route is the detonation of carbon-rich high explosives, which yields nanocarbons as a solid byproduct; the underlying condensation process also plays a crucial role in detonation modeling since the balance of performance and safety for organic chemical explosives favors materials that contain an excess of carbon, e.g. trinitrotoluene (TNT) and triaminotrinitrobenzene (TATB). The same process has long been believed to be possible in organic materials shock-compressed to high pressures, but experimental and simulation challenges have barred any conclusive study.
A Lawrence Livermore National Laboratory (LLNL) team has proven that nanocarbon can be synthesized by applying strong shocks to an organic material, and at the same time has provided insight into the physicochemical mechanisms controlling nanocarbon condensation at extreme conditions in a chemically reactive environment. The research is an important stepping stone for the improved characterization and modeling of organics and explosives exposed to strong shock waves and could open new avenues for nanocarbon synthesis. The work appears in Nature Communications.
The team focused on a prototypical carbon-rich system, liquid carbon monoxide (LCO) - first studied at Livermore almost four decades ago - and used laser-generated shock waves on nanosecond timescales, i.e., ultrafast compression. The same LLNL-developed technique was previously used to study other chemically reactive materials, but the current experiments were significantly more challenging, in part because of the cryogenic nature of LCO.
"Our experimental setup has allowed for the first time both the in-situ observation of nanocarbon precipitation and the recovery of nanocarbon samples," said LLNL scientist Mike Armstrong, who performed the ultrafast experiments.
The nanocarbon particles were further characterized using Transmission Electron Microscopy (TEM) and Raman spectroscopy and found to be similar with those recovered from detonation events. Large-scale atomistic molecular dynamics (MD) simulations performed on overlapping time-scales complemented the experimental results by supplying unprecedented detail of nanocarbon synthesis coupled with reactivity.
"The simulations were an important benchmark for our new, computationally efficient machine-learned reactive force-field, which we are now developing for application to other organic materials," said LLNL scientist Rebecca Lindsey, who carried out the MD calculations.
The research yielded a number of surprises, including the very short, subnanosecond timescale for the formation of the nanocarbon particles, and their largely classical growth mechanism under reactive conditions.
"High pressure reactivity is notoriously complex and is often modeled using empirical methods; by combining experiments and simulations we aimed to provide a better understanding of the fundamental processes involved and facilitate the development of broadly applicable modeling concepts for reactive systems at extreme conditions," said LLNL physicist Sorin Bastea, who led the Laboratory Directed Research and Development-funded project.
The research team also includes Nir Goldman, Michael Nielsen, Elis Stavrou, Larry Fried and Joe Zaug.