Researchers are getting better at growing miniature organs and embryos in test tubes or on chips. This creates new opportunities for answering research questions while reducing the need for animal testing in some cases.
Text: Anders Nilsson, first published in Medicinsk Vetenskap nr 2, 2025
The ability to cultivate cells in the laboratory has been significant for medical research and has led to several Nobel Prizes. Early attempts to sustain cells outside the body were made in the late 1800s, but the major revolution in this field came in 1951, when it became possible to sustain a human cell culture indefinitely. This discovery of the first so-called immortal cell line provided researchers with new opportunities to study and experiment on human biology. These cells were quickly involved in the development of the first polio vaccines in the 1950s and have since been used for everything from cancer research to virus mapping and testing new drugs.
Today, cell cultures are a standard tool in medical research, but they have their limitations. A plastic dish is not very similar to the cell's natural environment in the body, and growing in a thin layer on a culture plate is not like how cells organise themselves in tissues. This means that cultured cells lose certain natural functions and are not suitable for exploring all types of research questions.
To take advantage of the benefits of cell culture in more contexts, researchers have developed more advanced ways to cultivate living cells outside the body in recent years. The techniques vary, but a common feature is that the cultures have a more complex structure in three dimensions to better mimic natural tissue.
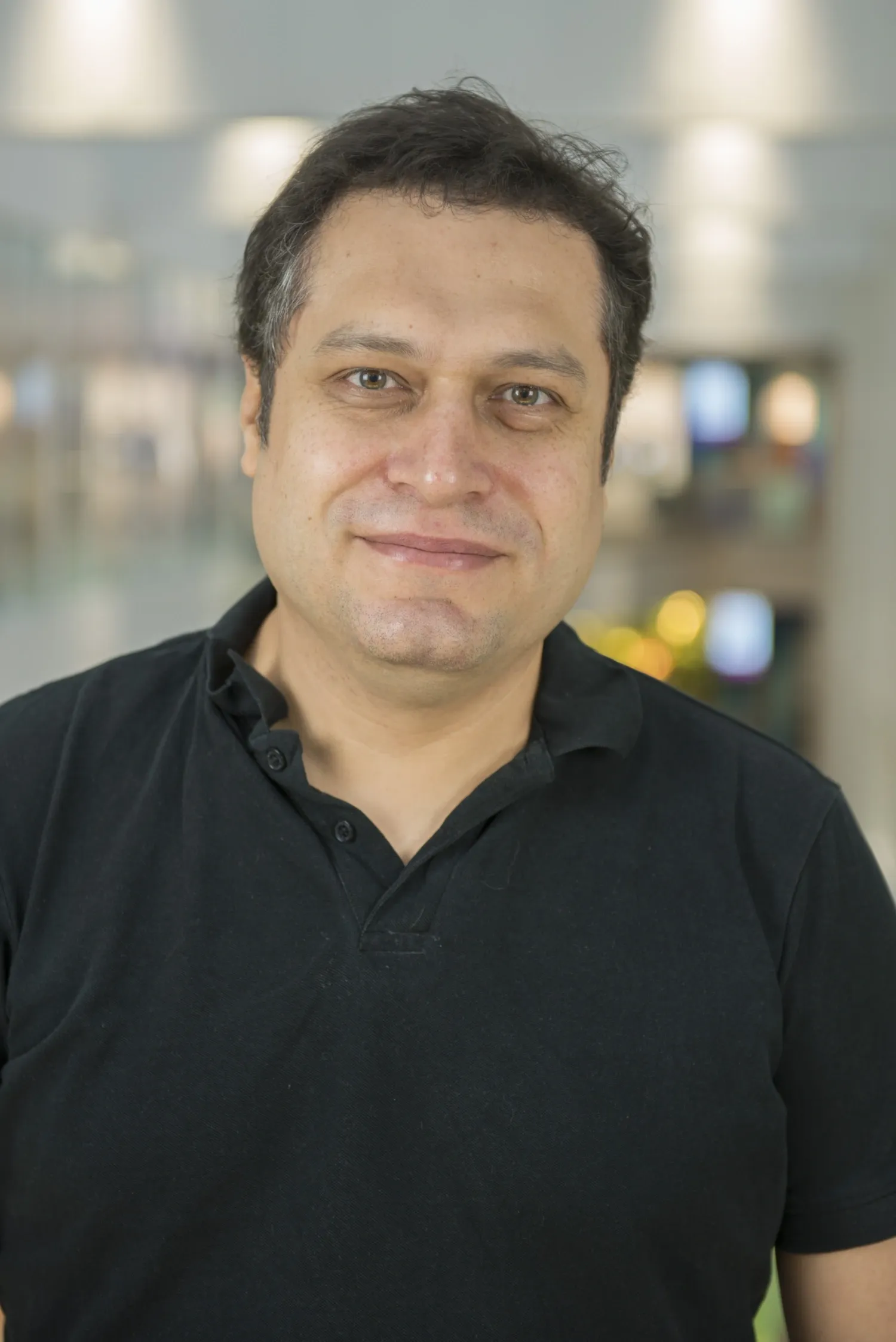
Mini-brains provide answers to research questions
Ivan Nalvarte researches Alzheimer's disease at the Department of Neurobiology, Care Sciences and Society, Karolinska Institutet. In his lab in the BioClinicum building, he cultivates human brain cells in small dishes. Under precisely the right conditions, brain cells can organise themselves into small clumps. The cells are active, connect with each other and start sending signals, just like cells in a real brain. A small piece of living brain tissue in a dish - the idea is staggering. But the small clusters do not think or feel anything, assures Ivan Nalvarte.
"It is neither possible nor relevant for us to cultivate a whole brain that would experience something," he explains. "We are interested in completely different questions," he says.
These small cell clusters are commonly referred to as mini-brains in popular science contexts. In the research world they are called cerebral organoids.For the past decade or so, they have been used worldwide, sometimes as an alternative to laboratory animals, to answer research questions about brain development, brain diseases or how the brain is affected by toxins or drugs. Similarly, organoids resembling other organs, such as lungs, liver and intestines, are used in other research.
Ivan Nalvartes' Alzheimer's research focuses on the significant sex-differences in the disease. Women are twice as likely as men to be affected, and researchers do not yet know why. He studies, among other things, how sex hormones like testosterone and oestrogen affect the risk of disease. Initially, he used mice, but this proved to be a difficult path forward.
"I realised that mouse models were not very suitable for us. Sex hormones affect the brain differently in humans and mice," he says.
Therefore, Ivan Nalvarte got the idea to try the new possibility of cultivating organoids. And, six years ago, he got started.
"The technique has been around for over a decade now, and there are protocols - descriptions of exactly how to do it - that are tested and reliable. "
We go to the lab, and from an incubator, he takes out a small plastic tray with six liquid-filled dishes. Tiny dots, 1-2 millimetres in diameter, float around in the pink nutrient solution. It is somewhat reassuring to see them in reality; it becomes immediately apparent that those small grains are not capable of thinking, dreaming or having anxiety.
In the microscope, the clusters appear slightly uneven, potato-shaped, and you can see a dark shadow in the centre of the larger ones. These are dead cells.
"As the organoids grow, the cells in the centre are too far from the surface. They suffer from nutrient and oxygen deficiency and die. Therefore, organoids are usually about this small. This can limit their use, but for us they do not need to be larger.
The advantages of organoids are many, he explains. First and foremost, it is about them being more realistic models of humans than regular cell cultures.
"Alzheimer's research often focuses on the plaque that forms in the brain during the disease. This is difficult to study in a normal cell culture, where the harmful substances are washed away by the nutrient solution. But in organoids, plaque forms similarly to a real brain.
Compared to laboratory animals, organoids are smaller, easier to handle, cheaper, more like humans, and a faster way to results.
Another important reason for Ivan Nalvarte is that experiments on organoids is preferable for ethical reasons. He has been involved in laboratory animal issues for a long time and has been a member of a national committee for laboratory animal protection since 2023.
"The EU has decided that animal testing should be phased out of European research in the long term, although no end date has been set. It will happen in many different ways, but the option of using organoids is definitely an important part," he says.
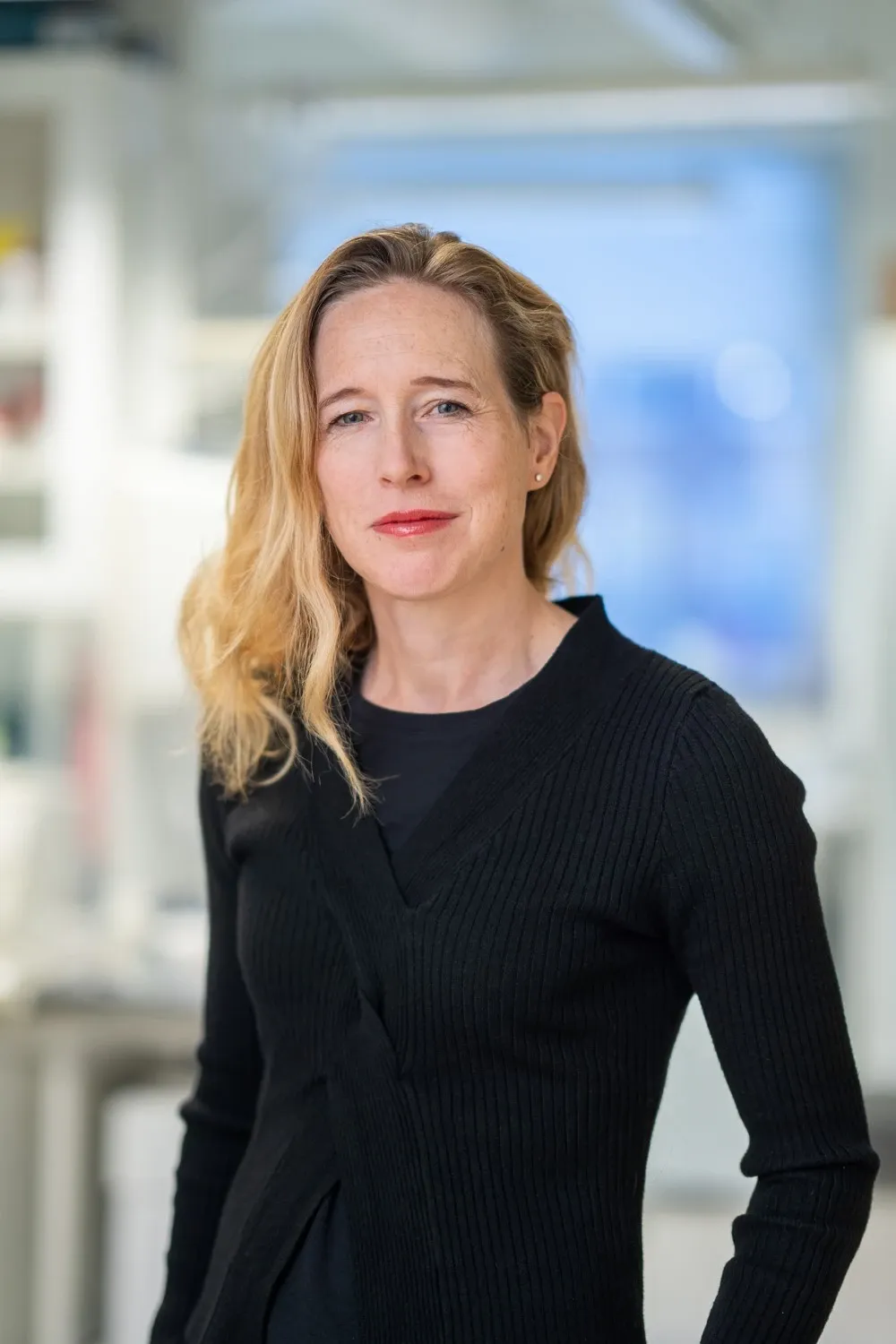
Organ on a chip mimics the environment inside the body
A stone's throw from Ivan Naljarte's workplace, Anna Herland conducts research at SciLifeLab in Solna. She is both a professor at KTH Royal Institute of Technology and a researcher at the Department of Neuroscience, Karolinska Institutet, and studies, among other things, what happens during brain haemorrhage in newborns - something that particularly affects very premature babies. She hopes that her research will lay the foundation for future treatments that can prevent permanent brain damage after such haemorrhages.
"Today, healthcare cannot prevent these injuries. It is thought that the damage is caused by the inflammation that occurs after the haemorrhage. That is why we want to investigate what happens if you block parts of the inflammatory reaction," she says.
But how do you study something like this? It is not possible to experiment on patients, and neither traditional cell cultures nor laboratory animals are suitable.
Anna Herland combines her technical and biomedical expertise to create a type of model commonly referred to as organ-on-chip. These are very small and meticulously controlled cell cultures in channels in a plastic tray.
"Organ-on-chip is not an entirely accurate name. It does not become like a whole organ on the chip, but it is a good technique for mimicking a certain function of an organ. Which is often exactly what we researchers need. The correct term - microfluidic-based cell culture - is quite complicated, so I understand why one wants to call it something simpler," she says.
Anna Herland brings out her collection of different types of chips. They are made of clear plastic and are the size of half a credit card. If you look closely, you can see small lines inside the plastic. These are the thin channels, a fraction of a millimetre wide. In them, she cultivates different types of cells to mimic conditions inside the body.
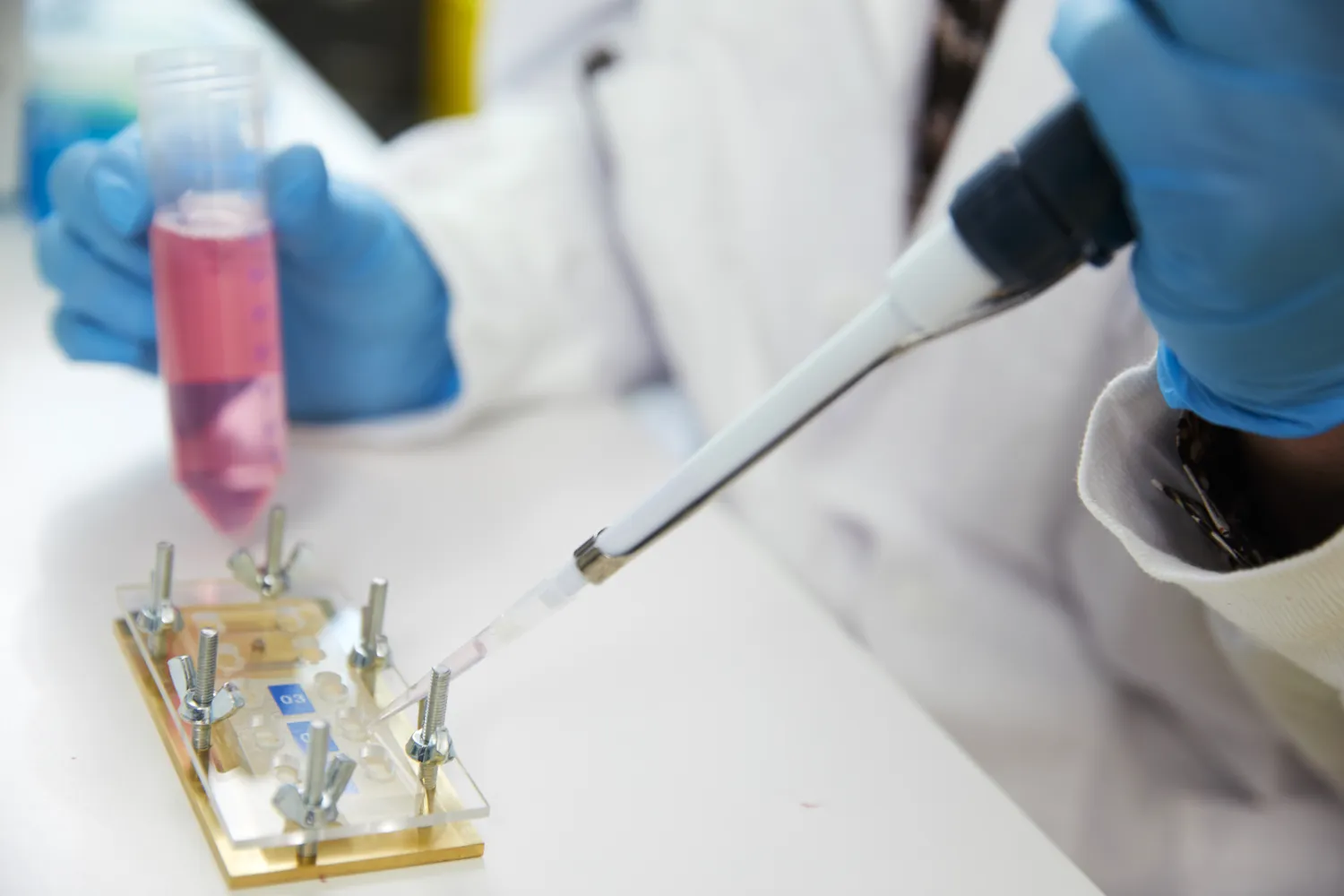
The great advantage of organ-on-a-chip is that several different cell types can be cultivated right next to each other, but at the same time separated. They get in contact with each other but are not forced into each other's environment.
"In the channels we can offer what suits each type: cells from the inside of the blood vessels are cultivated in a fast flow of a liquid that resembles blood, other cells that should have less access to nutrients and oxygen are cultivated in something more similar to the tissue fluid in our muscles," explains Anna Herland.
The presence of other cell types makes the cells function more normally, she explains.
"Everywhere in the body, there are thin surface layers consisting of one type of cell on top of another type. They only function normally when they interact with each other. This may involve how the blood vessel wall lets through nutrients or how the liver breaks down substances. Such things cannot be modelled in an regular cell culture with only one type of cells.
In addition to studying brain haemorrhage in newborns, Anna Herland also uses organs-on-chips to research certain metabolic diseases that cause brain damage. She has also developed a chip model of the blood-brain barrier, i.e. the brain's extra tight blood vessel walls. The model can be used for disease research or drug studies.
"Drug development is one of the major applications for organ-on-chips. They are used by many large pharmaceutical companies today, among other things as a replacement for animal testing. I believe that organ-on-chip was an important reason why the US has now dropped the requirement that drug trials on humans must be preceded by animal testing," she says.
A decade ago, when organ-on-chip was new, Anna Herland had to book herself into advanced clean rooms to access the expensive machines required to produce the chips. Today, her research group often manufactures their chips themselves in their own lab.
"We were lucky that the development of 3D printers took off around the same time as this research field emerged. It has made it much easier for us," she says.
Embryo models enable studies of early development
That every human being develops from a single cell has been known since the 1800s. But how it actually happens when the basic structure for a new human being arises from this single cell is still largely unknown. Science has been able to study the moment of fertilisation and the days that follow, but not what happens afterward, when the embryo attaches to the uterine wall and transforms from a homogeneous cell cluster to something heterogeneous and complex. The reason is, of course, that research on human embryos is strictly regulated. They cannot be kept alive longer than 14 days or returned to the uterus. And if it does not attach to the uterus around day seven, no further development occurs. Thus, research has practically been limited to the embryo's first week. What happens to the embryo during weeks 2-4 has therefore been shrouded in mystery. From week 5, research has had the opportunity to map the embryo's development by studying aborted foetuses.
However, in recent years, two important advances have provided new opportunities to study the early embryo. One is that researchers are no longer limited to real embryos from fertilised eggs but can cultivate embryo models. These are produced from stem cells, much like the brain organoids mentioned in the beginning of the article. The second advance is that it has become possible to get embryos and embryo models to attach to surface in the lab similar to a uterine wall. These advances have allowed researchers to study the embryo up to day 14.
This means that the period during which the embryo cannot be studied at all has shrunk to around two weeks: weeks 3 and 4.
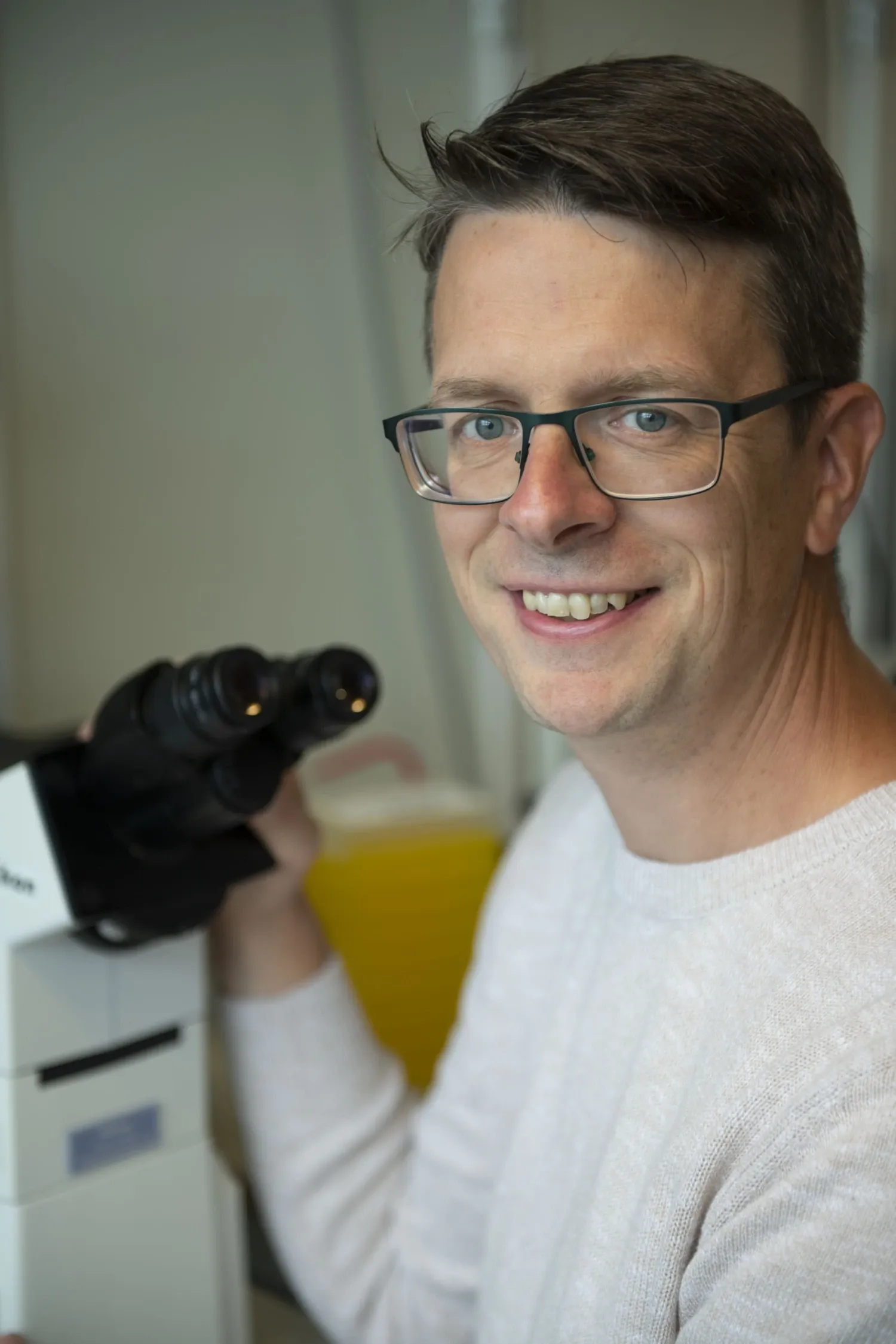
Fredrik Lanner researches early embryo development at the Department of Clinical Science, Intervention and Technology, Karolinska Institutet. He explains that the third and fourth weeks are among the most important and exciting events in the entire development of the foetus.
"This is when the body's basic cell types develop and the foundations for various organs are formed. Knowledge on how all this happens could lead to medical advances in several areas. It can help us understand the cause of miscarriages, infertility and birth defects," he says.
Knowledge of how different cell types arise could also lead to new types of treatments, where healthy cells that patients lack are produced in the lab.
"Imagine, for example, being able to produce insulin-producing cells for a patient with type 1 diabetes. Some colleagues in my research group are working on such matters."
The ability to work with embryo models offers several advantages for research. Unlike real donated embryos, models can be produced in large quantities. It is also possible to modify their genetic material to, for example, study the function of a particular gene.
"Our colleagues in other research groups here use embryo models to study how toxins and drugs affect embryos. It is a problem in healthcare today that pregnant women are prevented from taking many medications because it is not known whether the substances can harm the foetus," says Fredrik Lanner.
Embryo models are such a new phenomenon that Swedish law does not regulate how long they can be studied. But all parties agree that regulation would be desirable, and until it is in place, Fredrik Lanner is applying the 14-day rule even for models. The Swedish National Council on Medical Ethics has urged the Swedish government to regulate research on embryo models and to investigate whether research on real embryos should be allowed beyond day 14 - something that has been discussed in recent years, both in Sweden and internationally. 28 days is often mentioned as a possible new limit, but there are also other proposals, such as a flexible limit depending on the nature of the research.
"This is not a bad idea. But one good thing about the current legislation is its clarity. It is important that society has confidence in our research, that it does not seem unclear where the boundaries lie. That is why I still lean towards preferring a fixed limit of 28 days," says Fredrik Lanner.
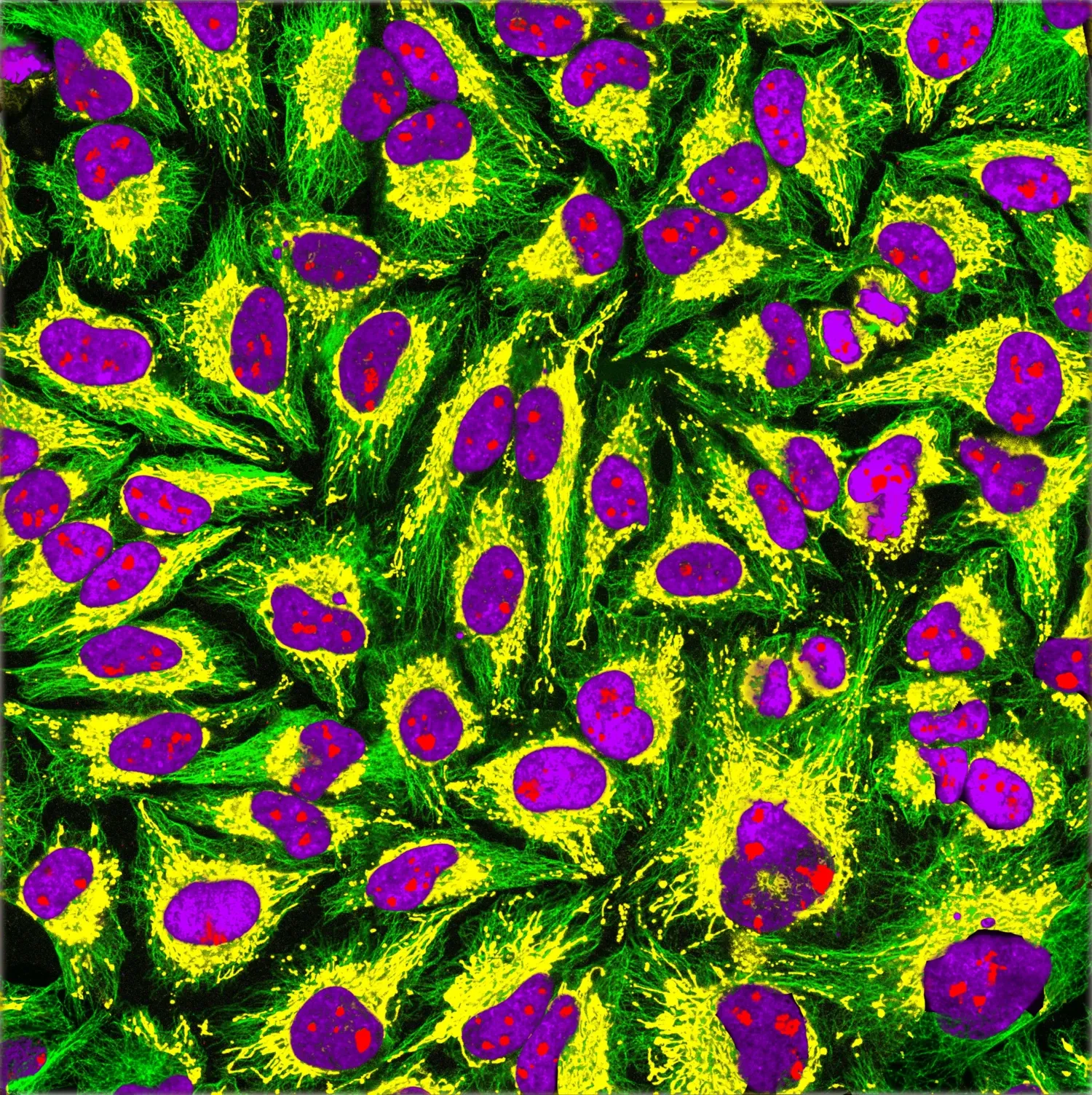
Henrietta Lacks' cells are cultivated all over the world
The first immortal cell line has been used in cell cultures all over the world since its discovery in 1951. These so-called HeLa cells have been of great benefit but are also a reminder of the importance of research ethics. They are named after Henrietta Lacks, the American cancer patient from whom the cells were originally taken, without her being informed or giving consent. The story of how her cells generated significant medical advances and economic value while her descendants lived in poverty and outside the American health insurance system gained widespread attention through the book The Immortal Life of Henrietta Lacks from 2010. The family has since received some compensation, as well as insight and influence in matters concerning her cells.
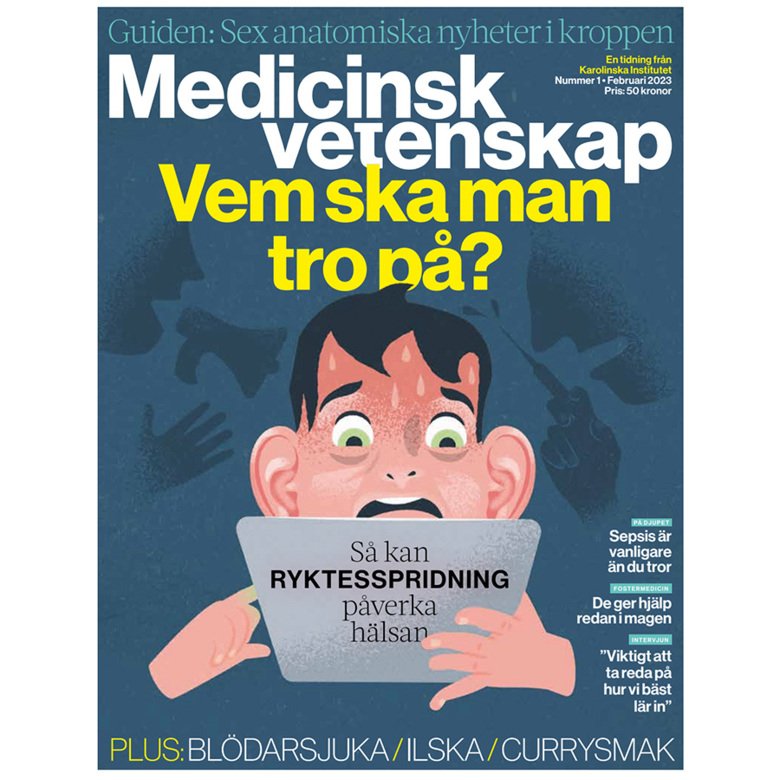
Läs fler spännande artiklar om medicinsk forskning
I Karolinska Institutets populärvetenskapliga tidning kan du läsa fler artiklar om det senaste inom medicinsk forskning. Bli prenumerant!